In today’s fast-paced world, the significance of portable electronic devices is undeniable. The gadgets like phones, laptops, smartwatches, or wireless headphones have seamlessly integrated into our lives. But this narrative doesn’t just end within these devices; it is intertwined with the extensive field of electric vehicles (EVs), sustainable energy storage solutions, and beyond.
As these dynamic markets evolve, Li-ion batteries have emerged as the crucial technology driving this tech revolution. Their lightweight, high-energy density and rechargeable nature have changed the industry, enabling longer-lasting, lighter, and more portable devices. Looking into the future, Li-Ion batteries stand as a vital enabler for sustainable transport and energy systems. Testament to this shift is the thriving electric vehicle market, which is expected to reach more than USD 800 billion by 2027. [1] These dynamics underscore the need to strengthen battery quality and safety across the spectrum, as every cell plays a key role.
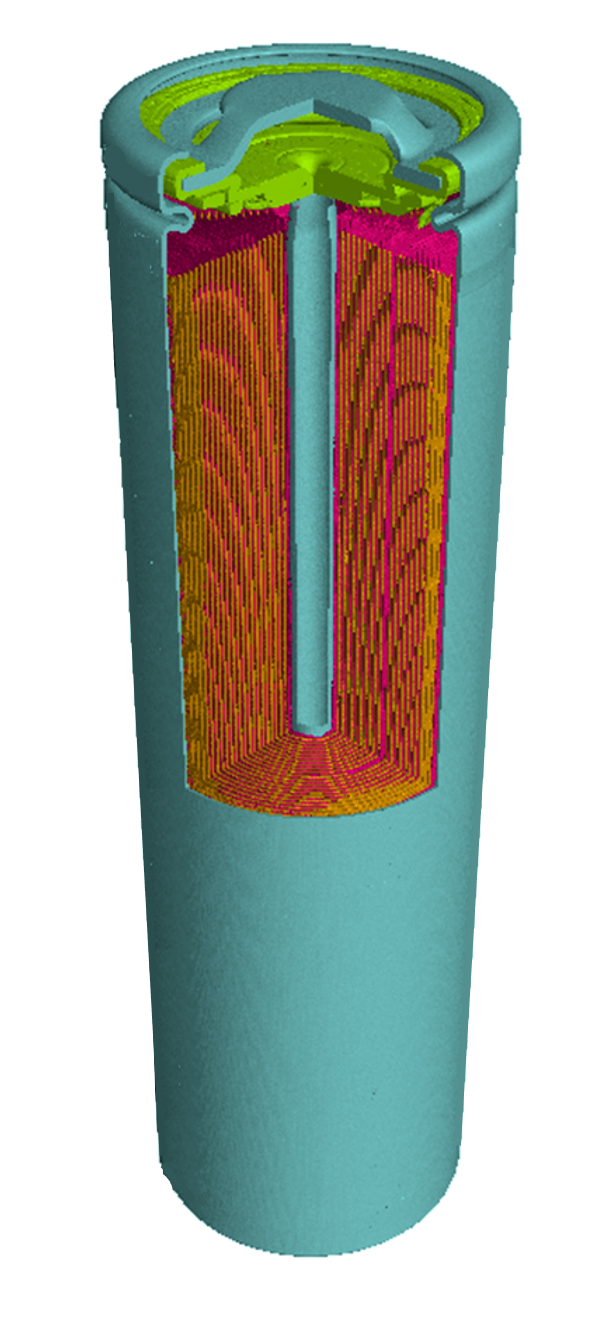
The use of X-ray computed tomography (CT) in analyzing Li-Ion batteries has become an integral aspect of their development, quality control, and safety assessment. CT allows us to peer beneath the surface harmlessly, without damaging the cell and identifying defects, irregularities, and structural weaknesses that might compromise the batterie’s reliable performance. The non-destructive nature of CT allows us to examine batteries in line, during the manufacturing process, or to monitor their performance degradation over their operational lifespan. [2] [3]
Utilizing CT it is possible to visualize the cell in multiple levels across various scales and resolutions, as illustrated in Figure 2– from macroscopic view of the whole cell and its casting to microscopic particles in a cathode. This versatile approach enables comprehensive battery analysis revealing all critical aspects hidden in dense components, e.g. housing pores, electrode layering, overhangs, particle defects or contaminations, and even documenting intricate processes like battery aging.
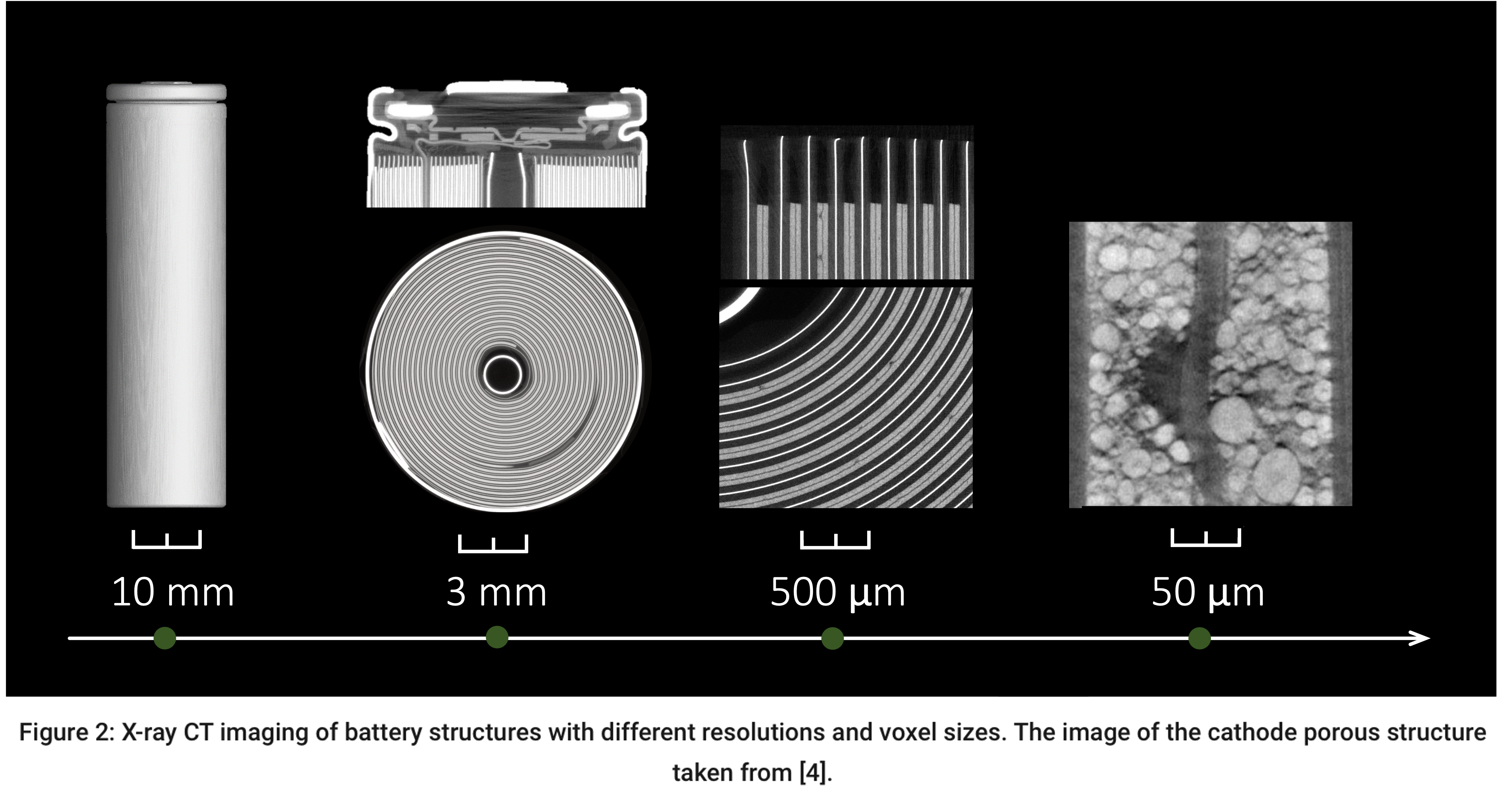
Battery cell imaging
A battery cell serves as the fundamental unit ensuring the overall structural integrity of the battery while securely containing the electrodes, electrolyte, and the chemical reaction inside. Given that the reaction leads to heat generation and material expansion, the arrangement of the battery components, cell sealing, and the arrangement of the cells into packs need to be controlled. This is where CT imaging steps in. CT is a suitable tool for the examination of these macroscopic parameters, on the centimeter and millimeter length scales. Furthermore, CT helps to identify any irregularities, such as inadequate sealing or foreign particles that may have infiltrated the seal during the manufacturing process. This inspection helps prevent potential loss of electrolyte, gas, or other substances that could lead to reduced battery efficiency, safety hazards, or premature failure. Moreover, CT enables precise measurements of cells, jellyroll structures, and more, ensuring meticulous manufacturing accuracy (see Fig.3).
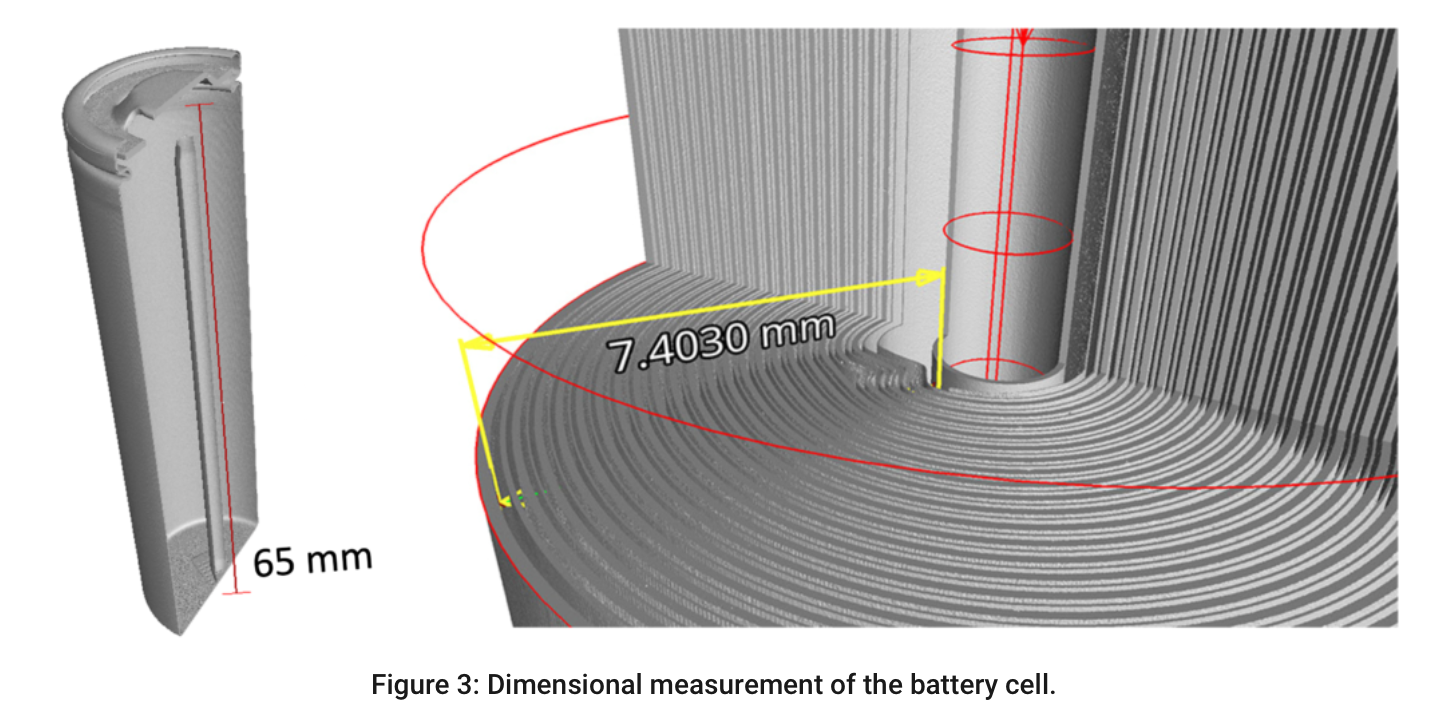
Active materials uncovered
All the essential electrochemistry for energy generation happens inside the cell – in the active layers of the battery. The repeating layers of cathode-separator-anode are affecting the energy storage and performance. Current collectors facilitate the efficient flow of electric current between the electrodes and the external circuit. These conductive materials are strategically positioned on the electrode surfaces to ensure efficient charge and discharge processes within the battery. All of these layers can be visualized, resolved, and separated using the CT technique (see Fig. 4). We can analyze the homogeneity and distribution of the active layers in the jellyroll. This ensures consistent energy distribution and storage within the electrodes, optimizing overall battery performance.
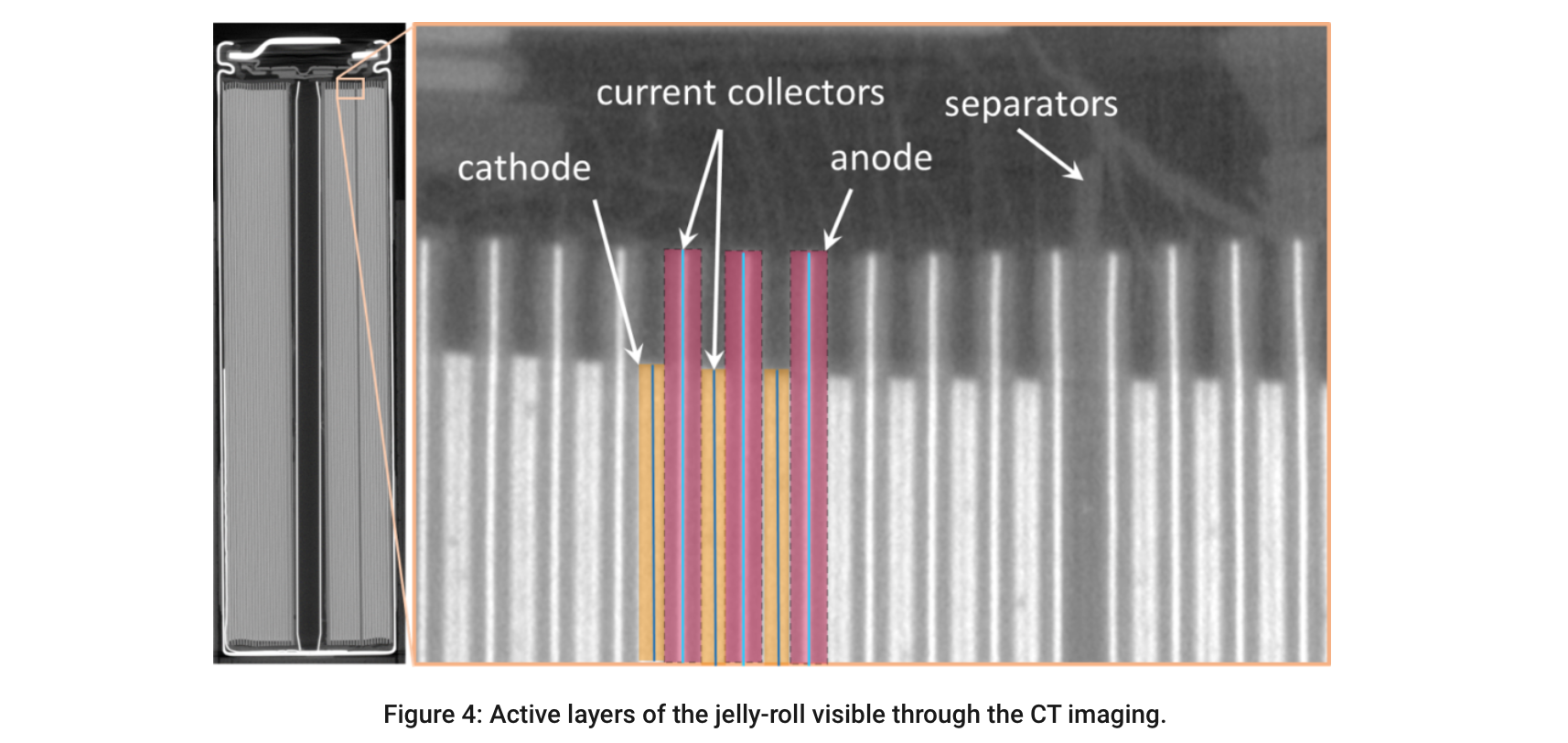
Furthermore leveraging the CT technology, we can examine potential variations in the anode overhang. The overhang area determines the inactive section of the lithium-ion battery, which plays a crucial role in preventing certain issues, such as lithium plating and dendrite formation. Maintaining balanced electrochemical processes requires the alignment and uniformity of the overhang to be ensured, a task that can be accomplished through CT analysis.
The quality of the electrodes themselves is highly important. The defects in the electrodes can lead to increased electrochemical impedance and polarization, acceleration of battery degradation, or even short circuits. [5] The application of CT imaging enables the identification of electrode defects (see Fig. 5), offering insights into their shapes and positions.
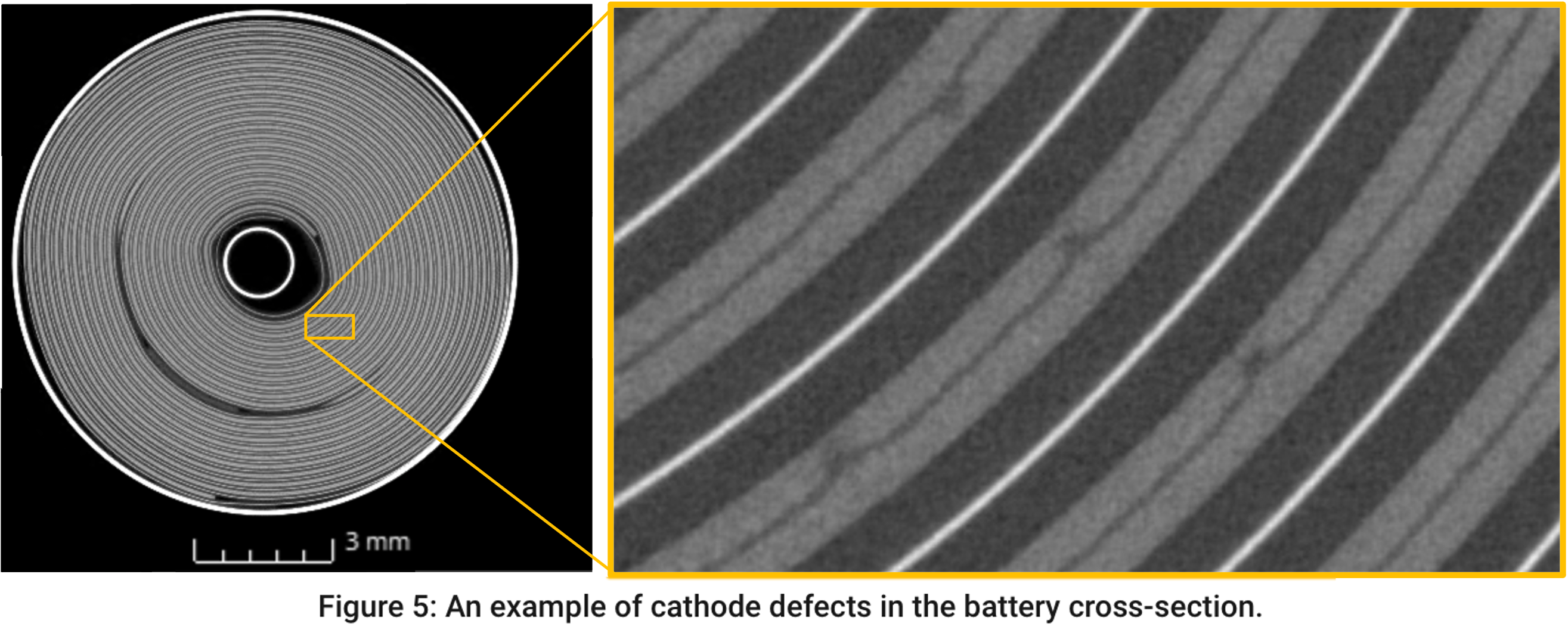
Moreover, utilizing submicron CT, defects can be examined in detail, while also enabling clear differentiation of the active particles within the cathode. The cathode has a typical porous structure that helps reduce the internal resistance of the battery. Electrons and ions can move more easily through the structure, enhancing conductivity and reducing energy losses during charge transfer. This results in improved battery efficiency and minimizes the impact of volume changes that occur during cycling. [6] With data analysis from submicron CT, we can analyze the volume distribution of the active particles (see Fig. 6) and characterize the particle structure. As a result, researchers and manufacturers can fine-tune electrode compositions, ultimately leading to more powerful, durable, and reliable batteries.
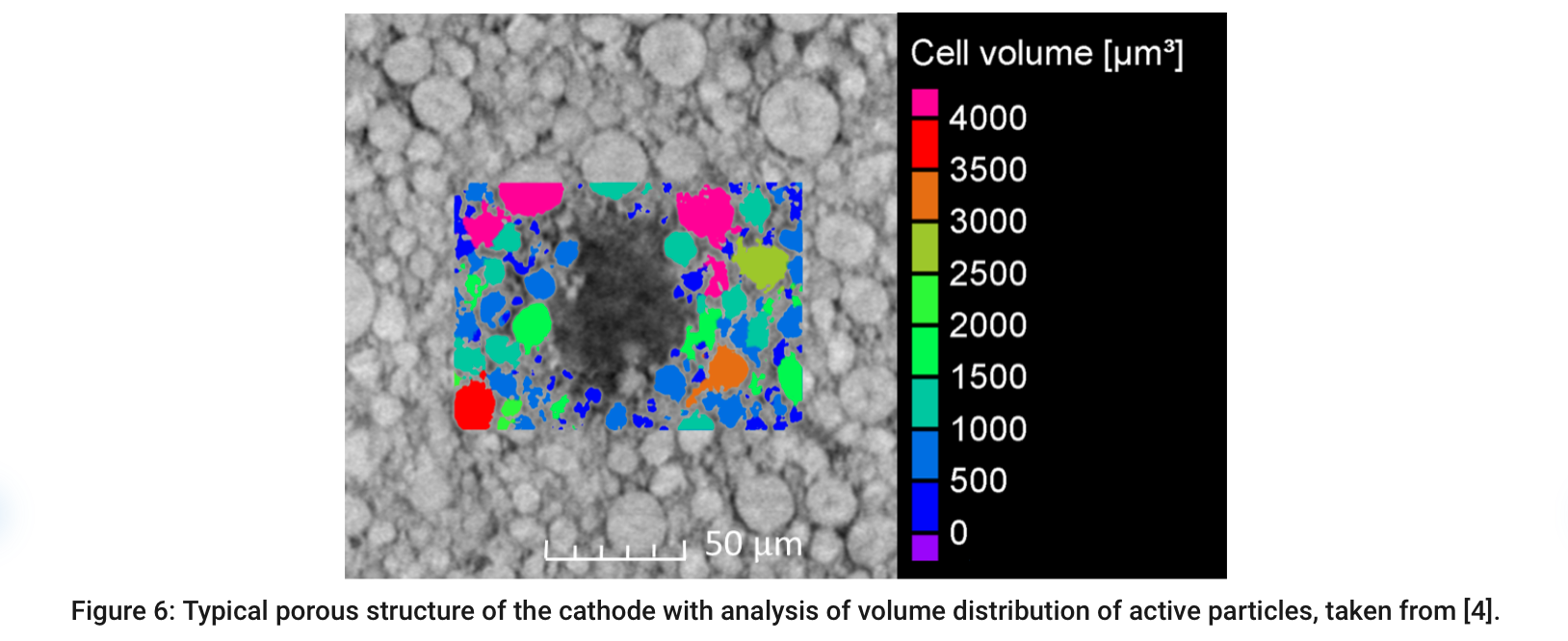
The impact of battery cycling
The aging of Li-Ion batteries is a complex process influenced by several factors. Over time and repeated charge-discharge cycles, these batteries experience gradual degradation in their capacity and performance. Elevated temperatures, high charge and discharge rates, as well as deep discharges, expedite this aging process. Due to the chemical reactions or material breakdown during cycling, the swelling of the electrodes occurs. The expansion can lead to various issues such as compromised structural integrity, decreased performance, and even safety hazards. Managing and mitigating battery aging is therefore essential to extend the lifetime and optimize the efficiency of Li-Ion batteries.
By offering detailed cross-sectional imaging, CT scans can provide insights into the internal changes within a battery. It can detect structural deformations, material shifts, and gas pockets that contribute to swelling. By scanning the batteries in different stages of their lifespan (see Fig. 7) it is possible to monitor the propagation of the deformations and analyze the durability of batteries.
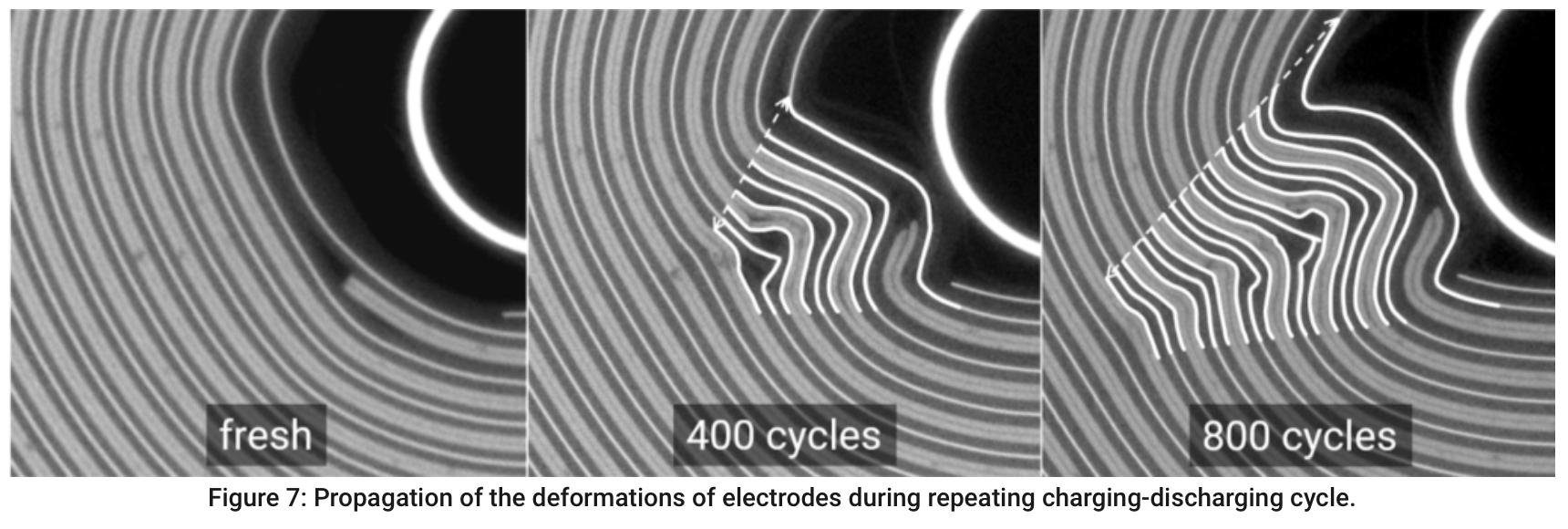
In conclusion, CT stands as an invaluable ally in the area of battery analysis, revealing hidden structures and processes behind the cell walls. Although our current technology has significantly broadened our understanding, CT battery analysis remains a dynamic field. The exploration of real-time imaging, in-situ analysis under diverse conditions, and deeper integration with computational simulations hold exciting possibilities in battery research and advancement.
If you’re intrigued by the potential of this technology, or if you seek to unlock the secrets within your batteries, we invite you to connect with us. Visit https://cactux.cz/ct-service/ to find our more about our possibilities.
Sources:
[1] Fully Charged. ZEISS eMobility Solutions from https://precise.zeiss.com/en/fully-charged
[2] Wu Y., Saxena S., Xing Y., Wang Y., Li C., Yung WKC, Pecht M. (2018). Analysis of Manufacturing-Induced Defects and Structural Deformations in Lithium-Ion Batteries Using Computed Tomography. Energies; 11(4):925. https://doi.org/10.3390/en11040925
[3] Pietsch P., Wood, V. (2017). X-Ray Tomography for Lithium Ion Battery Research: A Practical Guide. Annual Review of Materials Research. 47. 10.1146/annurev-matsci-070616-123957.
[4] Blažek P., Guricová P., Klvač O., Břínek, A., Kazda T., Zelenka F., Zikmund T., Kaiser J. ( 2021). Multiscale 3D Analysis of Defects and Temporal Development of Electrode Morphology in Lithium-Ion Batteries By X-Ray Computed Tomography. ECS TRANSACTION, p. 69 – 8, doi: 10.1149/10501.0069ecst;
[5] Qian G. et al. (2021). The role of structural defects in commercial lithium-ion batteries, Cell Reports Physical Science, Volume 2, Issue 9, 100554, ISSN 2666-3864, https://doi.org/10.1016/j.xcrp.2021.100554.
[6] Le Houx J., Kramer D., (2021). X-ray tomography for lithium ion battery electrode characterisation — A review, Energy Reports, Volume 7, Supplement 2, Pages 9-14, ISSN 2352-4847, https://doi.org/10.1016/j.egyr.2021.02.063.